Research Column
Author: Marc Kaufman
The experiment was hailed as a ground-breaking reproduction of how the essential components of life may have been formed, or at least a proof of concept that important building blocks of life could be formed from more simple components.
Little discussed by anyone outside the origins of life scientific community was that the experiment also produced a lot of a dark, sticky substance, a gooey tar that covered the beaker's insides. It was dismissed as largely unimportant and regrettable then, and in the thousands of parallel origins of life experiments that followed.
Today, however, some intrepid researchers are looking at the tarry residue in a different light.
Just maybe, they argue, the tar was equally if not more important as those prized amino acids (which, after all, were hidden away in the tar until they were extracted out.) Maybe the messy tar - produced by the interaction of organic compounds and an energy source - offers a pathway forward in a field that has produced many advances but ultimately no breakthrough.
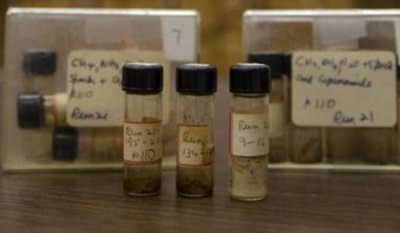
Those now studying the tar call their research "messy chemistry," as opposed to the "clean" chemistry that produced the acclaimed organic compounds.
There are other centers where different versions of "messy chemistry" research are under way - including George Cody's lab at the Carnegie Institution for Science and Nicholas Hud's at the Georgia Institute of Technology - but it is probably most concentrated at the Earth-Life Science Institute (ELSI) at the Tokyo Institute of Technology.
There, messy chemistry is viewed as an ignored but promising way forward, and almost a call to arms.
"In classical origin-of-life synthetic chemistry and biology you're looking at one reaction and analyzing its maximum result. It's A+B = C+D," said Irena Mamajanov, an astrobiologist with a background in chemistry, now a principal investigator at ELSI and head of the overall messy chemistry project.
"But life is not like that; it isn't any single reaction. They're looking at a subset of reactions and we ask: 'Why not look at the whole complex system?'"
There's a scientific lineage here - researchers have worked with complex systems and reaction systems in many fields, and in principle this is the same. It's taking a "systems" approach and applying it to that black box period on Earth when non-biological chemicals were slowly transformed (or transformed themselves) into chemical systems with the attributes of "life."
The messy chemistry work is getting noticed, and Mamajanov was a featured speaker on the "New Approaches to the Origins of Life" plenary at the 2017 Astrobiology Science Conference, in Mesa, Arizona. At ELSI alone, researchers have been working on messy chemistry using metals, using electricity, using radioactivity, using computational chemistry and using analytical chemistry to tease out patterns and structure in the tars.
Mamajanov says this messy chemistry approach - which she learned to some extent as a fellow at both Carnegie and Georgia Tech -- makes intuitive, as well as scientific sense because life is nothing if not complex.
Wouldn't it be logical for the origin of life to be found in some of the earliest complex systems on Earth, rather than in looking for straight-line processes that progress almost independent of all the chemistry happening around them?
It stands to reason that the gunky tar played a role, she said, because tars allow some essential processes to occur. Tars can concentrate compounds, can encapsulate them, and can provide a kind of primitive (messy) scaffolding that could eventually evolve into the essential backbones of a living entity.
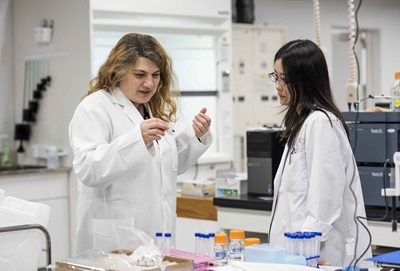
It's the structure, in fact, that stands out as a particularly promising aspect of messy chemistry. More traditional synthetic biology is looking for simple molecular structures created by clean reactions, while messy chemistry is doing the opposite.
The goal of messy chemists is to see what interesting chemical processes take place within a defined portion of the messy, complex sample. What unexpected, surprising compounds or chemical structures might be formed? And how might they shed light on the process of chemical self-organization and more generally the origin of life question?
In her lab on the basement floor of the ELSI main building, Mamajanov works with colleagues to synthesize her messy molecules and push further into understanding their structures, their potential ability to adapt, and their suitability as possible precursors to the RNA and DNA molecules that characterize life.
Her specific area of study is hyperbranched polymers - three-dimensional, tree-shaped chains of repeating molecules that connect with other similar molecules. The result is globular, presents multitudes of chemical reactions and has some hidden and protected spaces inside their globs. Related synthetic, or bio-mimicked chemicals (i.e., modeled on biological compounds and processes) have been used by the drug industry for some time.
With these hyperbranched polymers, Mamajanov has worked to produce pathways within the messy systems where the polymers show characteristics of evolvability.
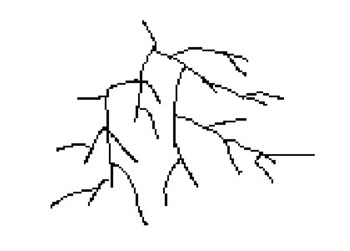
Her hyperbranched polymers are synthetic, as are those of noted synthetic chemists-in-search-of-biology such as Steven Benner, at the Foundation for Applied Molecular Evolution and Gerald Joyce of the Scripps Institute.
But the starting points are quite different, as are the goals. The two men are working to create clean chemical systems that produce the building block molecules that they want, but without the tar. Mamajamov is intentionally making tar.
Eric Smith, a specialist in complexity systems, physics and chemistry who is also at ELSI sees the messy approach as containing the seeds of an important new way forward. "What is now called messy chemistry used to be completely out of the mainstream," said Smith. "That is no longer the case."
Smith described how John Sutherland of the Laboratory of Molecular Biology at Cambridge, U.K. won accolades for his work on the prebiotic assembly of important building blocks for RNA, using controlled chemistry that avoided all the messiness.
But he was also criticized later for using a such a controlled model - early Earth, after all, did not have any outside controller - and Smith said Sutherland is incorporating the messier side of prebiotic chemistry today, although tar remains an enemy rather than a potential friend.
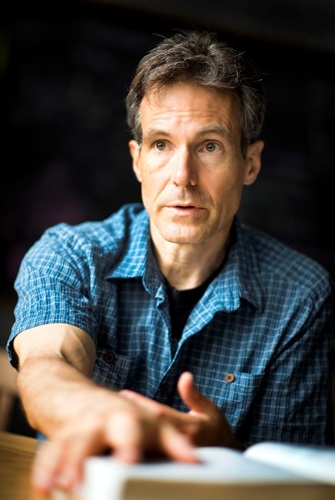
"Now he's going back to a one pot synthesis, allowing reactions that would have to be less controlled than what he was doing before," Smith said of Sutherland. "He may do it in a way quite different from Irena and others involved in messy chemistry, but it seems to allow for many more complex reactions."
And complexity is indeed the desired endpoint. Not simply repetitive reactions and not random ones, but rather reactions that are very complex but ultimately structured.
This is where another novel aspect of the messy chemistry approach comes into play: Mamajanov and others at ELSI are collaborating with practitioners of "artificial chemistry," computer simulated versions of what could be happening in messy interactions.
The work is being done primarily by Nathaniel Virgo, an artificial life specialist who uses computing to learn about how chemical systems behave once you leave the laboratory world where the number of chemical components is small and controlled.
And his big question: "Are there situations in which you can get 'order from disorder' in chemistry - to start with a messy system and have it spontaneously become more ordered? If so, what kinds of conditions are required for this to happen, and what kinds of ordered states can result?
Mamajanov needs Virgo's computations to analyze and project forward what a messy chemical system might do. And Virgo needs the messy chemistry as a test bed of sorts for his abstracted questions about, in effect, making order out of what appears to be chaos. They are, for each other, hypothesis-generating machines.
Virgo pointed to several primary reasons why computational work is important for answering the question of creating order from disorder (and ultimately, he is convinced, life from non-life.)
"The first is simply that studying messy chemistry experimentally is really hard. If you have a test tube containing a mess, it takes a lot of work to find out what molecules are in it, and basically impossible to know what reactions are happening, at least not without an enormous amount of work. In contrast, in a simulation you know exactly what molecules and reactions are present, even if there are millions of different types."
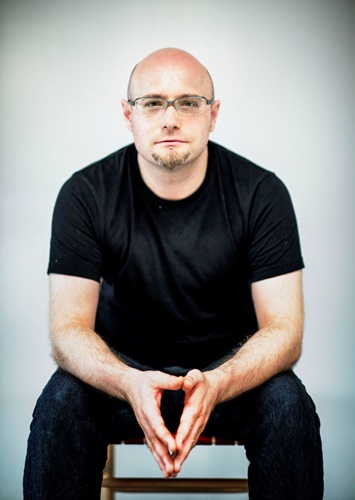
The second reason involves the fundamental issue of studying specific chemical systems versus studying general mechanisms.
"As a complex systems scientist, I first want to know what, in general, is required, for a given phenomenon to occur. Once this is known, it should become clear which real systems will exhibit the right kinds of properties.
"This allows us to narrow down the vast space of possible hypotheses for the origins of life, rather than simply testing them one at a time. It should also give us some insight into the question of whether life might be possible with completely different kinds of chemistry than the protein-nucleic acid-metabolite chemistry we have on Earth.
From his studies he has found that in messy chemical systems, chemical self-production occurs and that the systems can change dramatically in response to small changes such as an increased temperature.
"This suggests that messy chemistry is fundamentally qualitatively different from clean chemistry - adding more species doesn't just mean the system gets harder to study, it also means that fundamentally new things can happen."
And in the origin of life world, things are happening.